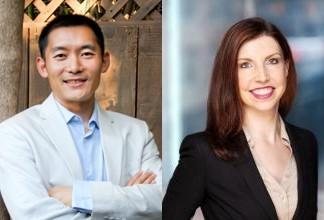
Christopher Cheng, Ph.D., and Valerie Merkle, Ph.D.
This is the last of five short articles on the importance of cardiovascular device biomechanical compatibility. These articles discuss: 1) why biomechanical compatibility is important, 2) the role of biomechanical compatibility in the device development process, 3) fatigue evaluation for initiating clinical trials, 4) fatigue evaluation for market approval, and 5) the future of biomechanical compatibility assessment.
Fracture Does Not Equal Failure
There is a tendency for negative visceral reaction when the topic of device fracture arises. Indeed, device fracture is usually an indication of fatigue failure; however, it has been shown that fracture does not always lead to clinical sequelae. In fact, some devices are designed to preferentially fracture as part of their normal operation, and others that do not fracture may actually indicate excessive stiffness and be the cause of clinical consequences (Figure). Therefore, fracture, defined as breaking a continuous structure into discontinuous portions, is not necessarily a sign of impending adverse clinical outcomes or even a design flaw. Sometimes, the problem can be fixed just by understanding the underlying vascular biomechanics and altering intervention strategies. For example, in the case of femoropopliteal artery stents, the incidence of device fracture was dramatically reduced by avoiding placing stiffer stents in regions susceptible to extreme kinking at the adductor hiatus near the knee. Even well-designed devices can fracture and may contribute to subsequent clinical sequelae. However, the overall benefits to patients may still far outweigh the risks of fracture.
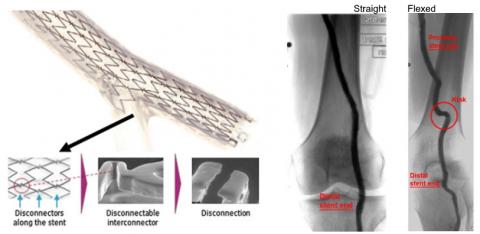
Figure. Left: The Stentys coronary bifurcation stent was designed to fracture at certain bridges in order to open up into a branch ostium (adapted from Laborde et al., Eurointervention 2007, 3(1):162-165 and Mishra, Indian Heart J, 68(6):841-850). Right: Some peripheral stents exhibit high axial and bending stiffness which can cause arterial kinking distal to the stent in the femoropopliteal artery due to knee flexion, which can lead to thrombosis or restenosis (adapted from Gokgol et al., Biomech Model Mechanobiol 2019, 18(6):1883-1893).
Understanding Will Lead to Better Products
The impact of biomechanical compatibility is usually only revealed over the long term, requiring clinical studies and a deep understanding of how biomechanics affect biology and clinical outcomes. However, there is no debate that increased understanding of these interactions will lead to more precise product development, which should yield better devices with less ambiguity during the development cycle. One of the mechanisms for this is more precise and comprehensive standards and guidance for biomechanical evaluation. For example, while there is guidance about radial pulsatile testing in arteries, there is a dearth of consensus about axial, bending, crush, and twisting deformations for structural heart, coronary, aortic, peripheral artery, venous, and neurovascular devices.
Biomechanical Compatibility Is in Its Infancy
Evaluation of fatigue (how the dynamic anatomy affects the device) is often treated like an inconvenient but necessary portion of device development, and biomechanical harmony (how the device affects the dynamic anatomy) is rarely considered. There is good reason for this lack of attention: biomechanics is not enticing. Would an engineer rather be acknowledged for inventing and designing a novel device, or merely for confirming that that device will survive for 10 years in vivo? Probably the former. Would a company rather invest in producing more products that can generate more revenue, or spend time and money optimizing biomechanical compatibility? Definitely the former. However, it is now recognized that biomechanical compatibility is critical to device design, evaluation, and ultimately patient safety. As an industry, we are just beginning to pay attention to biomechanical compatibility and learn how it impacts device performance and clinical outcomes.
A Call to Action
As some device categories become more mature, we should be working together as an industry to develop more defined guidance around biomechanical compatibility, including loading conditions, computational modeling, and physical testing. For newer device categories, especially for those addressing lower-risk patients who may be healthier, more active, and younger, we must challenge the conventions of using “normal” loading conditions for 10 years of life. For example, replacement heart valves are already tested for 15 years, and as iliofemoral vein stents and structural heart devices get used on healthier, younger patients, perhaps 20-30 years of cycling in more compliant vessels is more appropriate. This can be accomplished either at each respective manufacturer or external to the manufacturer, potentially through non-profit organizations such as Cardiovascular Implant Durability (cvidconference.org). Furthermore, understanding the dynamic behavior of blood vessels goes far beyond medical devices. Measurement of vascular motion can be a critical diagnostic tool. Vessel elasticity and motion, for example, have been shown to correlate with cardiovascular health, and may play roles in diagnostics, treatment planning, and development of pharmaceutical or genetic therapies.
Partnering with Regulatory Bodies
To further the field and include regulatory considerations, it is helpful to include the FDA and other regulatory bodies in industry-wide efforts. For example, when communication between regulators and companies is collaborative, device fractures identified during a pivotal study may not preclude commercial approval. Consideration is given to the severity, location, frequency, timing, and clinical relevance of fractures, as well as the overall benefit-risk profile of the device for its labelled indication. Manufacturers should work collaboratively with regulators when fractures are first observed in order to ensure study subjects are adequately protected through root cause analyses. Manufacturers and regulators have the same ultimate goal: to provide patients with timely access to safe and effective medical devices.
About the Authors
Dr. Christopher Cheng has 20+ years of experience in academic research and the medical device industry, spanning hemodynamics, vascular motion, device design, manufacturing, preclinical testing, clinical trials, and marketing. He is considered the preeminent expert in vascular motion, having over 100 publications and edited Handbook of Vascular Motion (PROSE Book Award Nominee, https://www.elsevier.com/books/handbook-of-vascular-motion/cheng/978-0-1...), the first and only book dedicated to how blood vessels move. Dr. Cheng runs the Global Science & Technology – Medical Division (gst.com/med), the first dedicated organization to help medical device companies holistically evaluate and improve biomechanical compatibility of medical implants. He is also an Adjunct Professor in the Division of Vascular Surgery at Stanford, Director of the Vascular Intervention Biomechanics & Engineering (VIBE) lab (vibelab.stanford.edu), and Director of the Cardiovascular Implant Durability Conference (cvidconference.org). Previously, Dr. Cheng was co-founder and CEO of Kōli, Inc., an early-stage medical device company developing a catheter-based solution for gallstone disease. Dr. Cheng studied BME and EE at Duke University, earned his Master’s and Ph.D. in Biomechanics at Stanford University, and currently serves as a board member of the Duke University Pratt School of Engineering. Email: winecpc@gmail.com
Dr. Valerie Merkle is the Associate Director of Regulatory Strategy at Syntactx. With the Syntactx Team, she provides expert assistance to clients seeking regulatory approvals and product adoption worldwide. Prior to joining Syntactx, Dr. Merkle was a leader in the U.S. Food and Drug Administration (FDA) in the Center for Devices and Radiological Health (CDRH) vascular and endovascular devices team. In her role, she managed and reviewed over 650 complex regulatory submissions, including pre-submissions, investigational device exemptions, as well as 510(k) and PMA marketing submissions. Her FDA experience included managing challenging submissions for first-of-a-kind devices and those with unique benefit/risk profiles, providing her the opportunity to serve as the FDA lead to a meeting of the Circulatory System Devices Panel. Her additional expertise and outreach include standards work, cardiovascular materials research, and FDA/external research collaborations. Dr. Merkle is a Steering Committee Member for the Greenberg Stent Summit, a unique conference that brings together representatives from industry, clinical practice, and FDA to discuss current issues in endovascular interventional therapy. Dr. Merkle holds a Bachelor of Science in Chemical Engineering from Bucknell University, a Ph.D. in Biomedical Engineering from the University of Arizona, and is an Innovation Fellow of the Fogarty Institute. She has co-authored numerous peer-reviewed manuscripts and has presented at multiple academic, scientific, and technical conferences.